Theoretical cosmology and elementary particle physics
The Theoretical Cosmology and Elementary Particle Physics group comprises researchers studying a diverse set of topics in particle physics, gravitational physics, and cosmology. This includes studying the dark energy and dark matter in the Universe, building new models of space and time, and predicting the behavior of fundamental particles in models of physics beyond the standard model. Some of the group’s research involves numerical simulation using some of the world’s largest supercomputers. This group is always looking for talented undergraduates to join them and participate in original research into the fundamental nature of the matter and forces that govern our universe. This group also brings their research into the classroom, teaching courses on modern topics in relativity, cosmology, and particle physics.
Cosmology
Cosmology is the study of the universe. Over the past decade, observational cosmology has provided us with an accurate, new and surprising description of the evolution of the universe. These data have highlighted existing issues and raised entirely new questions regarding the microphysical processes behind these macroscopic phenomena.
At Syracuse we are actively involved in research into most aspects of modern cosmology and its connections to particle physics. We are studying the earliest phenomena in the universe; inflation, the generation of density perturbations, the origin of dark matter, baryogenesis and the cosmic microwave background radiation. We are also deeply involved in investigating the origin of the recently observed acceleration of the universe.
For more detailed descriptions of our research interests, see the research page of Scott Watson.
Elementary Particle Physics
One of the great triumphs of twentieth century physics was the elucidation of the structure of matter and the forces that govern it. The language describing this subnuclear world is the language of quantum field theory. Field theory is central to understanding the extreme quantum and relativistic phenomena at this level of structure. Our improved understanding of field theory has also provided us with the tools to go beyond the standard model. Its interrelation with mathematical ideas from modern geometry and topology have broadened our horizons and led to physics beyond the Standard Model. Supersymmetry and string theory have deep and important roots in our modern understanding of field theory. Physicists at Syracuse are actively engaged in consolidating and extending our understanding of this level of structure in our world using quantum field theory and its modern developments.
Lattice Field Theory
Lattice Field Theory Quantum field theory has proven a very successful theoretical framework for understanding the interactions of elementary particles. One very convenient formulation of such theories is given by the so-called path integral prescription originally due to Richard Feynman. Within this formalism one can compute the vacuum expectation values of arbitrary products of fields at different spacetime points by integrating them over a distribution given by the exponential of a function of the fields called the action. For more information, please see the web page of Dr. Simon Catterall or contact Dr. John "Jack" Laiho.
Quantum Computing and Quantum Information Science
Recently there has been a surge of interest in problems that lie at the interface between fundamental physics and quantum information science. The last two decades have seen the development of the new field of quantum information science, which analyses how quantum systems may be used to store, transmit and process information. The field encompasses a broad swathe of science and engineering and is poised to revolutionize much of science and technology with the development of practical quantum computing.
Within the arena of theoretical physics, quantum computing offers the potential to calculate for the first time the properties of strongly interacting dense matter such as found in neutron stars or high temperature superconductors. Very recently there have also emerged surprising and deep connections between black holes, quantum gravity and quantum error correction.
One specific new idea that has arisen in this context is the possibility of reformulating (lattice) quantum field theory as a tensor network. Tensor network formulations have the potential to avoid the notorious sign problem that plagues Monte Carlo methods of simulating quantum field theory and explicitly reveals connections to holographic approaches to quantum gravity. Such approaches also can serve as the first step in a reformulation of QFTs suitable for quantum computation. Profs. Simon Catterall and Jay Hubisz are pursuing research in this area.
Faculty
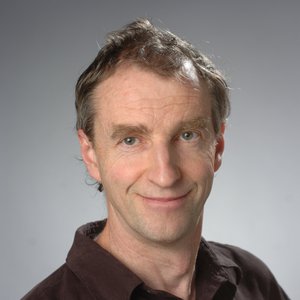
Simon Catterall
Professor
Email: smcatter@syr.edu
Phone: 315.443.5978
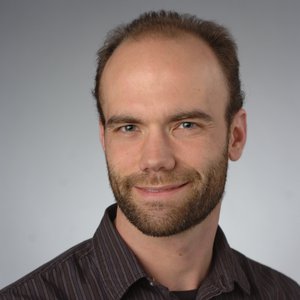
Jay Hubisz
Professor
Email: jhubisz@syr.edu
Phone: 315.443.2653
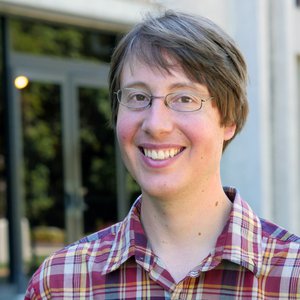
John "Jack" Laiho
Associate Professor
Email: jwlaiho@syr.edu
Phone: 315.443.3469
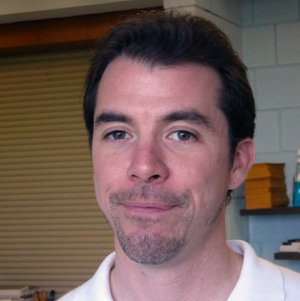
G. Scott Watson
Professor
Email: gswatson@syr.edu
Phone: 401.225.1177