Close Encounters of the Supermassive Black Hole Kind: Tidal Disruption Events and What They Can Reveal about Black Holes and Stars in Distant Galaxies
Astrophysicists from Syracuse University and the University of Leeds collaborate with high school students in Syracuse to confirm the accuracy of an analytical model that can unlock key information about supermassive black holes and the stars they engulf.
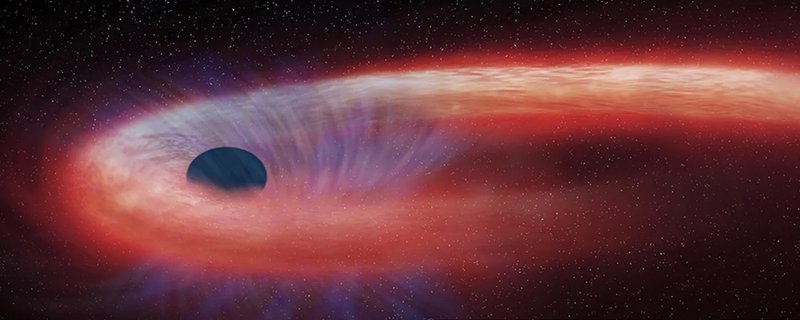
Artist's representation of a tidal disruption event (a star being torn apart by a black hole). Credit- NASA / CXC / M. Weiss
At the center of most large galaxies lives a supermassive black hole (SMBH). The Milky Way has Sagittarius A*, a mostly dormant SMBH whose mass is around 4.3 million times that of the sun. But if you look deeper into the universe, there are vastly larger SMBHs with masses that can reach up to tens of billions of times the mass of our sun.
Black holes grow in mass by gravitationally consuming objects in their near vicinity, including stars. It’s a catastrophic and destructive end for stars unlucky enough to be swallowed by SMBHs, but fortunate for scientists who now have an opportunity to probe otherwise-dormant centers of galaxies.
TDEs Light the Way
As the name implies, black holes do not emit any light of their own, making them very difficult for researchers to observe. But when a star comes sufficiently close to a supermassive black hole, it can be destroyed by the black hole’s immense tidal gravitational field through an interaction that is, effectively, an extreme instance of the Earth’s tidal interaction with the Moon. Some of the tidally destroyed material falls into the black hole, creating a very hot, very bright disk of material as it does so. This process, known as a tidal disruption event (TDE), provides a light source that can be viewed with powerful telescopes and analyzed by scientists.
TDEs are relatively rare - predicted to take place roughly once every 10,000 to 100,000 years in a given galaxy. One to two dozen TDEs are typically detected annually, but with the advent of new technology like the Vera C. Rubin Observatory, currently under construction in Chile, hundreds are anticipated to be observed in the coming years. These powerful observatories scan the night sky for rising and falling sources of light, and thus “survey” the cosmos for time-changing astronomical phenomena. Using these surveys, astrophysicists can perform studies of TDEs to estimate the properties of SMBHs and the stars that they destroy. One of the things that researchers try to understand is the mass of both the star and the SMBH. While one analytical model has been used quite often, a new one was recently developed and is now being tested.
The Advent of Analytical Models
The accretion rate – or rate at which a star’s stellar material falls back onto the SMBH during a TDE – reveals important signatures of stars and SMBHs, such as their masses. The most accurate way to calculate this is with a numerical hydrodynamical simulation, which uses a computer to analyze the gas dynamics of the tidally destroyed material from a TDE as it rains onto the black hole. While precise, this technique is expensive and can take weeks to months for researchers to compute one TDE.
In recent decades, physicists have devised analytical models to calculate the accretion rate. These models present an efficient and cost-effective method for understanding the properties of disrupted stars and black holes, but uncertainties remain about the accuracy of their approximations.
A handful of analytical models currently exist, with perhaps the most well-known being the “frozen-in” approximation; this name derives from the fact that the orbital period of the debris that rains onto the black hole is established, or “frozen-in,” at a specific distance from the black hole called the tidal radius. Proposed in 1982 by Lacy, Townes and Hollenbach, and then expanded upon by Lodato, King and Pringle in 2009, this model suggests that the accretion rate from massive stars peaks on a timescale that can range from one to 10 years depending on the mass of the star. This means that if you’re looking at the night sky, a source could initially brighten, peak, and decline with time over timescales of years.
A New Way Forward
Eric Coughlin, a physics professor at Syracuse University, and Chris Nixon, associate professor of theoretical astrophysics at the University of Leeds, proposed a new model in 2022, simply referred to as the CN22 model, which determines the peak timescale for TDEs as a function of the properties of the star and the mass of the black hole. From this new model, they recovered TDE peak timescales and accretion rates that agreed with the results of some hydrodynamical simulations, but the broader implications of this model – and also its predictions over a wider range of stellar type, including the mass and age of the star – were not completely elucidated.
To better characterize and understand the predictions of this model in a wider context, a team of researchers from Syracuse University, led by Ananya Bandopadhyay, a Ph.D. student in the Department of Physics, conducted a study to analyze the implications of the CN22 model and test it against different types of stars and SMBHs of various masses. The team’s work has been published in Astrophysical Journal Letters. In addition to lead author Bandopadhyay, co-authors included Coughlin, Nixon, undergraduate and graduate students from the Department of Physics, and Syracuse City School District (SCSD) students. The SCSD students’ involvement was made possible through the Syracuse University Research in Physics (SURPh) program, a six-week paid internship where local high schoolers engage in cutting-edge research alongside faculty and students from the College of Arts and Sciences’ Department of Physics.
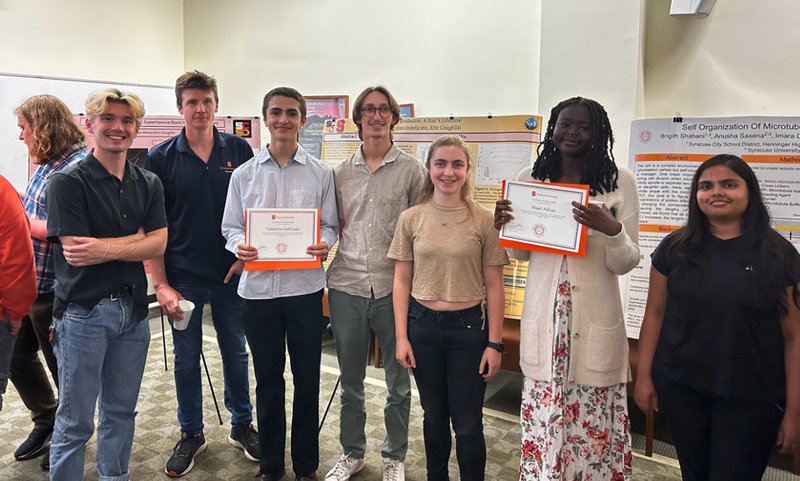
Syracuse City School District students who took part in the SURPh program served as co-authors on the study. From left to right are Matt Todd (physics graduate student), Eric Coughlin (SU physics professor), Valentino Indelicato (SURPh participant), Dan Paradiso (physics graduate student), Julia Fancher (physics undergraduate student), Aluel Athian (SURPh participant) and Ananya Bandopadhyay (physics graduate student and lead author).
During the summers of 2022 and 2023, the SCSD students collaborated with Syracuse physicists on computational projects that tested the validity of the CN22 model. They used a stellar evolution code called ‘Modules for Experiments in Stellar Astrophysics’ to study the evolution of stars. Using these profiles, they then compared the accretion rate predictions for a range of stellar masses and ages for the “frozen-in” approximation and the CN22 model. They also performed numerical hydrodynamical simulations of the disruption of a sun-like star by a supermassive black hole, to compare the model predictions to the numerically obtained accretion rate.
Their Findings
According to Bandopadhyay, the team found that the CN22 model was in extremely good agreement with the hydrodynamical simulations. Moreover, and perhaps most profound, was the finding that the peak timescale of the accretion rate in a TDE is very insensitive to the properties (mass and age) of the destroyed star, being ~ 50 days for a star like our Sun destroyed by a black hole with the mass of Sagitarrius A*.
Most striking and surprising about this result is that the “frozen-in” model makes a very different prediction. According to the “frozen-in” model, the same TDE would produce an accretion rate that would peak on a timescale of two years, which is in blatant disagreement with the results of hydrodynamical simulations.
“This overturns previously held notions about the way that TDEs work and what types of transients you could possibly produce by totally destroying a star,” says Bandopadhyay. “By confirming the accuracy of the CN22 model, we offer proof that this type of analytical method can greatly speed up the inference of observable properties for the disruption of stars having a range of masses and ages.”
Their study also addresses another previous misconception. By clarifying that complete TDEs cannot exceed month-long timescales, they disprove the earlier belief that they can be used to explain long-duration light curves that peak and decay on multiple-year spans. In addition, Coughlin notes that this paper verifies that peak fallback rate is effectively independent of the mass and age of the disrupted star and is almost entirely determined by the mass of the SMBH, a key indicator that models like CN22 can help researchers constrain masses of SMBHs.
“If you measure the rise time, what you could be directly peering into is actually the property of the supermassive black hole, which is the Holy Grail of TDE physics - being able to use TDEs to say something about the black hole,” says Coughlin.
Acknowledging the paper’s influence on the field, Bandopadhyay was invited by the American Astronomical Society to give a presentation of the team’s findings at the society’s 243rd meeting in New Orleans on January 11, 2024.
Looking to the future, the team says by confirming the accuracy of the CN22 model, this study opens a window for researchers to make observable predictions about TDEs, which can be tested against existing and upcoming detections. Through collaboration and ingenuity, researchers at Syracuse are bringing details about the physics of black holes to light and helping explore areas of the distant universe that were once untraceable.