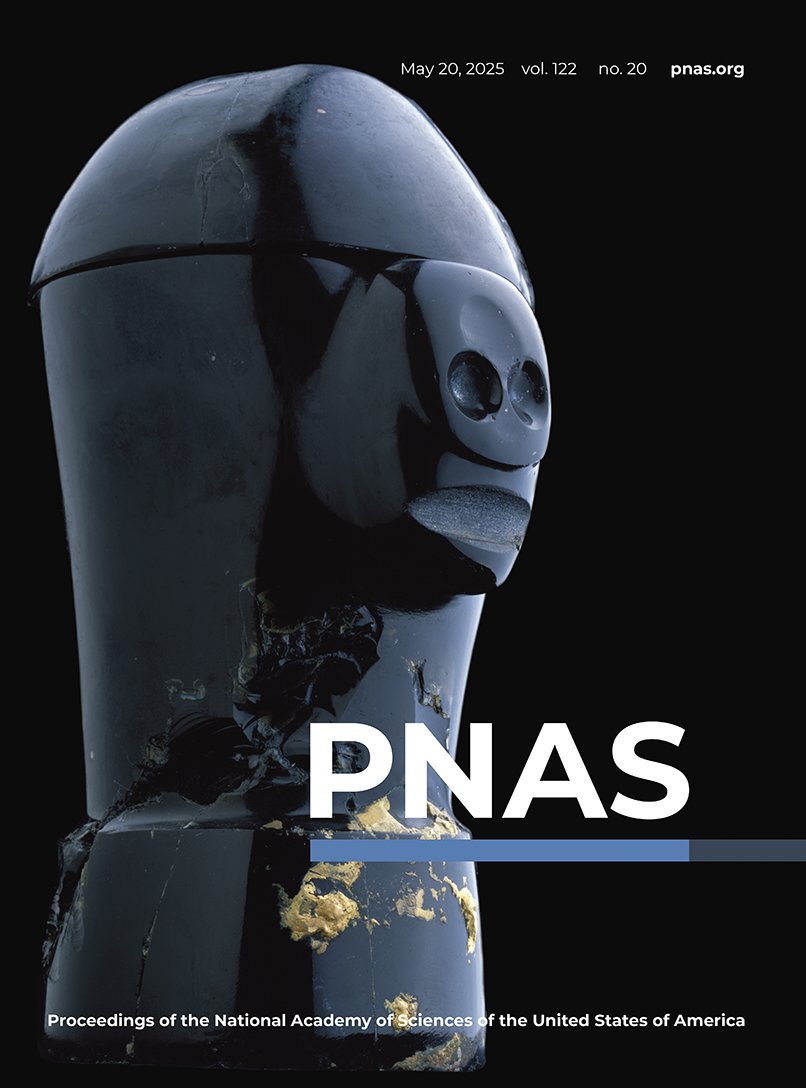
A team of researchers from Syracuse University and MIT published a study in PNAS uncovering evidence that oxygenation in the ocean—crucial for life as we know it—may have occurred earlier than previously thought, offering new insights into the evolution of our planet.
Key Takeaways:
- The Great Oxidation Event (GOE) (~2.4 billion years ago) was a major turning point in Earth’s history, marking the first significant rise of oxygen in the atmosphere—transforming Earth from an oxygen-free world into one capable of supporting complex life.
- A New Study in Proceedings of the National Academy of Science (PNAS) reveals that the aerobic nitrogen cycle in the ocean may have occurred about 100 million years before oxygen began to significantly accumulate in the atmosphere, based on nitrogen isotope analysis from ancient South African rock cores.
- These findings not only refine the timeline of Earth’s oxygenation but also highlight a critical evolutionary shift, where life began adapting to oxygen-rich conditions—paving the way for the emergence of complex, multicellular organisms like humans.
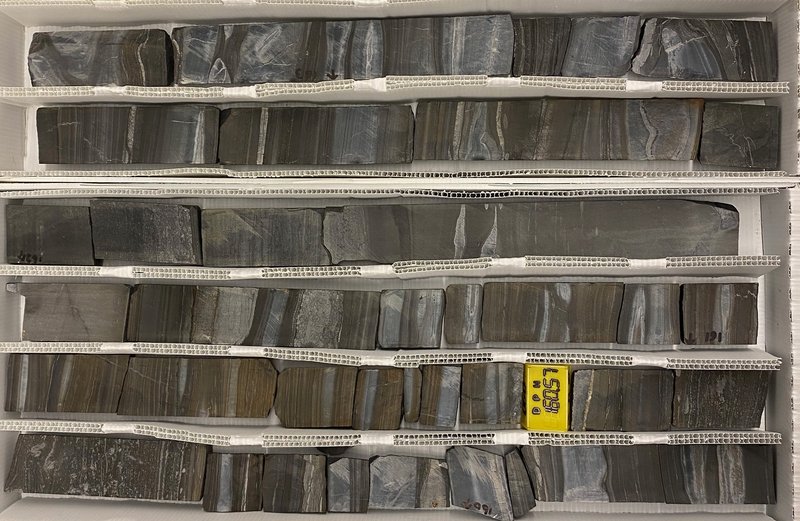
By delving into ancient rock records, scientists have uncovered new clues that sharpen the timeline of Earth’s oxygenation—shedding fresh light on one of the most transformative chapters in our planet’s evolutionary story. (Courtesy: Benjamin Uveges)
Several key moments in Earth’s history help us humans answer the question, “How did we get here?” These moments also shed light on the question, “Where are we going”? – offering scientists deeper insight into how organisms adapt to physical and chemical changes in their environment. Among them is an extended evolutionary occurrence over 2 billion years ago, known as the Great Oxidation Event (GOE). This marked the first time that oxygen produced by photosynthesis—essential for the survival of humans and many other life forms—began to accumulate in significant amounts in the atmosphere.
If you traveled back in time to before the GOE (more than ~2.4 billion years ago), you would encounter a largely anoxic (oxygen-free) environment. The organisms that thrived then were anaerobic, meaning they didn’t require oxygen and relied on processes like fermentation to generate energy. Some of these organisms still exist today in extreme environments such as acidic hot springs and hydrothermal vents.
The GOE triggered one of the most profound chemical transformations in Earth’s surface history. It marked the transition from a planet effectively devoid of atmospheric oxygen—and inhospitable to complex life—to one with an oxygenated atmosphere that supports the biosphere we know today.
Scientists have long been interested in pinpointing the timing and causes of major shifts in atmospheric oxygen because they are fundamental to understanding how complex life, including humans, came to be. While our understanding of this critical period is still taking shape, a team of researchers from Syracuse University and MIT is digging deep—literally—into ancient rock cores from beneath South Africa to unearth clues about the timing of the GOE. Their work provides new insight into the pace of biological evolution in response to rising oxygen levels—and the long, complex journey toward the emergence of eukaryotes (organisms whose cells contain a nucleus enclosed within a membrane).
The study, published in the journal Proceedings of the National Academy of Science, was led by Benjamin Uveges ’18 Ph.D., who completed the project as a postdoctoral associate at MIT and collaborated with Syracuse University Earth sciences professor Christopher Junium on the chemical analyses.
Answers Embedded in Rock
To step back in time, the research team analyzed sedimentary rock cores collected from several sites across South Africa. These locations were carefully selected because their rocks, dating back 2.2 to 2.5 billion years, fall within the ideal age range for preserving evidence of the GOE. By analyzing stable isotopic ratios embedded in these rocks, the team uncovered evidence of oceanic processes that required the presence of nitrate – an indicator of more oxygen-rich conditions.
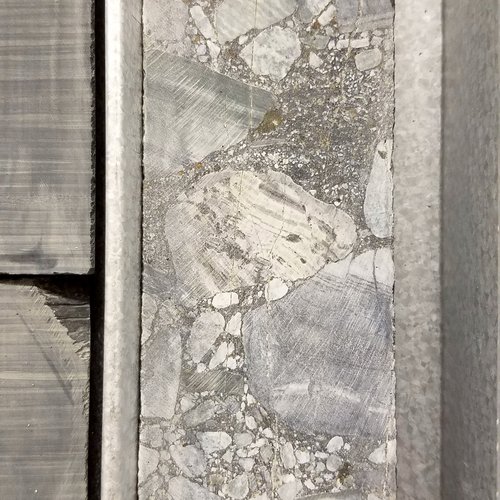
To analyze the ancient sediment, Uveges worked with Junium, an associate professor of Earth and environmental sciences at Syracuse University. Junium specializes in studying how past environments evolved to better understand future global change. His state-of-the-art instruments were essential for obtaining accurate readings of trace nitrogen levels.
“The rocks that we analyzed for this study had very low nitrogen concentrations in them, too low to measure with the traditional instrumentation used for this work,” says Uveges. “Chris has built one of only a handful of instruments in the world that can measure nitrogen isotope ratios in samples with 100 to 1,000 times less nitrogen in them than the typical minimum.”
In Junium’s lab, the team analyzed nitrogen isotope ratios from South African rock samples using an instrument called an Isotope Ratio Mass Spectrometer (IRMS). The samples were first crushed into powder, chemically treated to extract specific components, then converted into gas. This gas was ionized (turned into charged particles) and accelerated through a magnetic field, which separated the isotopes based on their mass. The IRMS then measured the ratio of ¹⁵N to ¹⁴N, which can reveal how nitrogen was processed in the past.
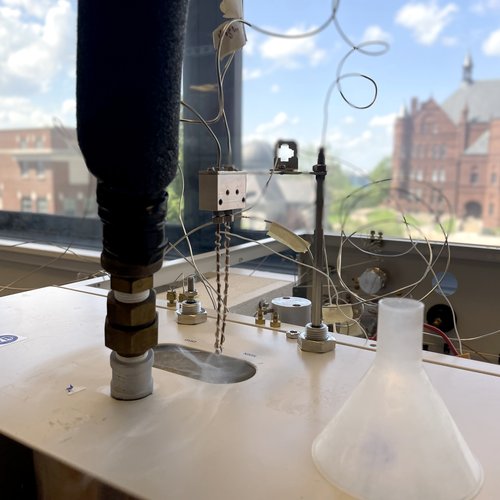
So how does this process reveal past oxygen levels? Microbes (short for microorganisms) influence the chemical makeup of sediments before they become rock, leaving behind isotopic signatures of how nitrogen was being processed and used. Tracking changes in ¹⁵N to ¹⁴N over time helps scientists understand how Earth’s environment, particularly oxygen levels, evolved.
Rewriting the Oxygen Timeline
According to Uveges, the most surprising finding is a shift in the timing of the ocean’s aerobic nitrogen cycle. Evidence suggests that nitrogen cycling became sensitive to dissolved oxygen roughly 100 million years earlier than previously thought – indicating a significant delay between oxygen buildup in the ocean and its accumulation in the atmosphere.
Junium notes that these results mark a critical tipping point in the nitrogen cycle, when organisms had to update their biochemical machinery to process nitrogen in a more oxidized form that was harder for them to absorb and use.
“All of this fits with the emerging idea that the GOE was a protracted ordeal where organisms had to find the balance between taking advantage of the energy gains of oxygenic photosynthesis, and the gradual adaptations to dealing with its byproduct, oxygen,” says Junium.
As oxygen produced through photosynthesis began to accumulate in the atmosphere, this rise in oxygen led to the extinction of many anaerobic organisms and set the stage for the evolution of aerobic respiration—a process that uses oxygen to break down glucose and provides the energy needed for functions like muscle movement, brain activity and cellular maintenance in humans and other animals.
“For the first 2 plus billion years of Earth’s history there was exceedingly little free oxygen in the oceans or atmosphere,” says Uveges. “In contrast, today oxygen makes up one fifth of our atmosphere and essentially all complex multicellular life as we know it relies on it for respiration. So, in a way, studying the rise of oxygen and its chemical, geological and biological impacts is really studying how the planet and life co-evolved to arrive at the current situation.”
Their findings reshape our understanding of when Earth's surface environments became oxygen-rich after the evolution of oxygen-producing photosynthesis. The research also identifies a key biogeochemical milestone that can help scientists model how different forms of life evolved before and after the GOE.
“I hope our findings will inspire more research into this fascinating time period,” says Uveges. “By applying new geochemical techniques to the rock cores we studied, we can build an even more detailed picture of the GOE and its impact on life on Earth.”
This work was funded by grants including: An NSF CAREER award (Syracuse University - Christopher Junium) and a Simons Foundation Origins of Life Collaboration award (MIT – Benjamin Uveges, Gareth Izon and Roger Summons).
Citation: B.T. Uveges, G. Izon, C.K. Junium, S. Ono, & R.E. Summons, Aerobic nitrogen cycle 100 My before permanent atmospheric oxygenation, Proc. Natl. Acad. Sci. U.S.A. 122 (20) e2423481122, https://doi.org/10.1073/pnas.2423481122 (2025).