Scientists Spin Up a New Way to Unlock Black Hole Mysteries
Syracuse University astrophysicist co-authors a study in Nature that details how observations of a wobbling disk following a tidal disruption event can be used to estimate black hole spin.
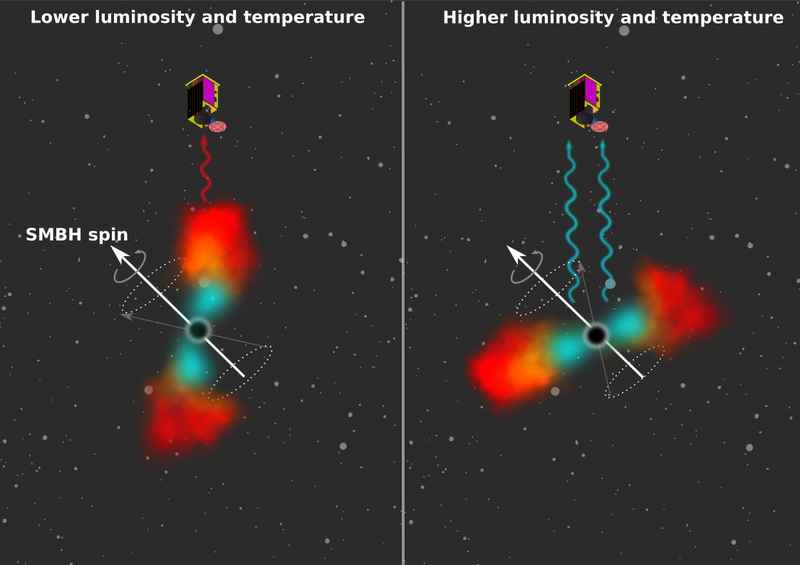
Figure depicting the precession (movement of the rotational axis) of an accretion disk formed from the debris of a disrupted star. The left panel shows the precession phase when the accretion disk is close to an edge-on configuration, which results in the smaller disk area being observed and thus lower luminosity. The observer can see mostly the colder, outer parts of the precessing disk. The right panel depicts a nearly face-on precession phase, when the visible disk area is larger and hence the luminosity also increases. The inner, warmer parts of the disk are then fully exposed. (Credit: Michal Zajaček and Dheeraj R. “DJ” Pasham)
Black holes are among the most studied but least understood cosmic phenomena for astrophysicists. While not technically a "hole," these objects derive their name from the fact that nothing, including light, can escape the grasp of their immense gravitational field. While black holes do not emit light of their own, any gas in their immediate vicinity gets very hot and luminous as it spirals into the event horizon – the distance from the hole at which the gravitational field is so immense that light cannot escape – and this gas can be episodically supplied when a black hole feeds on a star.
When a star comes sufficiently close to a supermassive black hole (SMBH) it is pulled apart. Some of the tidally destroyed material falls into the black hole, creating a very hot, very bright disk of material called an accretion disk before it plunges through the horizon. This process, known as a tidal disruption event (TDE), provides a light source that can be viewed with powerful telescopes and analyzed by scientists.
Among the physicists who study TDEs to learn more about SMBHs is Eric Coughlin, a professor at Syracuse University’s College of Arts and Sciences. He was part of a seminal study in 2023 with Dheeraj R. “DJ” Pasham, a research scientist at MIT, and Thomas Wevers, who at the time was a Fellow of the European Southern Observatory. They proposed a model for a repeating partial TDE, which is when a star is captured by a SMBH, but instead of being completely destroyed, the high-density core of the star survives, allowing it to orbit the black hole more than once. Their results were the first to use a detailed model to map a star’s surprising return orbit about a supermassive black hole – revealing new information about one of the cosmos’ most extreme environments.
A Wobbling Source
Following up on that research, the team of scientists joined collaborators from several leading institutions, including NASA, Masaryk University in the Czech Republic, Tel Aviv University, the Polish Academy of Sciences, and elsewhere, to explore a different TDE phenomenon. This time they zeroed in on the wobbling stellar material of an accretion disk present in the aftermath of a TDE. They focused their research on a TDE called AT2020ocn, which came onto the scene in February 2020 when a bright flash emanating from a galaxy about a billion light years away was spotted by the Zwicky Transient Facility in California.
Using NASA’s NICER telescope, the team collected observations of AT2020ocn over 200 days following its initial detection. They discovered that the event emitted X-rays that appeared to peak every 15 days, for several cycles, before eventually petering out. They interpreted the peaks as times when the TDE’s accretion disk wobbled face-on, emitting X-rays directly toward NICER’s telescope, before wobbling away as it continued to emit X-rays (similar to waving a flashlight toward and away from someone every 15 days).
By examining the wobbling disk following a TDE, researchers believe it will allow them to make novel insights about spin rates of black holes. Their results, published in the journal Nature, mark the first time that scientists have used observations of a wobbling disk following a tidal disruption event to estimate black hole spin.
An Evolutionary Tool
During a TDE, scientists predict that a star may fall onto a black hole from any direction, generating a disk of white-hot, shredded material that could be tilted, or misaligned, with respect to the black hole’s spin. (Imagine the accretion disk as a tilted donut that is spinning around a donut hole that has its own, separate spin.) As the disk encounters the black hole’s spin, it wobbles as the black hole pulls it into alignment. Eventually, the wobbling subsides as the disk settles into the black hole’s spin. Scientists predicted that a TDE’s wobbling disk should therefore be a measurable signature of the black hole’s spin.
“The spin of a supermassive black hole tells you about the history of that black hole,” says Pasham, the study’s lead author.
As new telescopes such as the Rubin Observatory come online in the coming years, Pasham foresees more opportunities to study the spin rates of black holes.
“Even if a small fraction of those that Rubin captures have this kind of signal, we now have a way to measure the spins of hundreds of TDEs,” he says. “Then we could make a big statement about how black holes evolve over the age of the universe.”
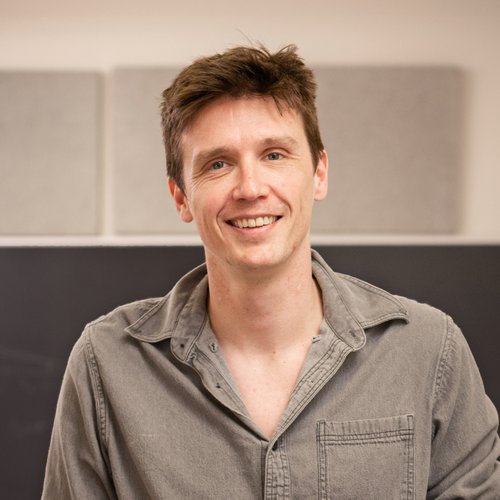
Syracuse University physicist Eric Coughlin was involved in understanding the properties of the accretion flow that formed around the black hole during this TDE, the radius and mass of the star, and the mass and spin of the SMBH. Because the spin of black holes can be modified by how they accrete from their environment, Coughlin notes that this study fills in another piece of the puzzle when it comes to understanding the evolution and behavior of black holes. For example, if many of the black holes in the universe are spinning very rapidly, it suggests that material is consistently funneled onto a black hole from the same direction over cosmological timescales. If, on the other hand, black holes are not all rapidly rotating (or very few are), then it suggests that black holes grow intermittently and in a sporadic way.
“Which one of these processes occurs is tied to galaxy formation and evolution, and hence measuring black hole spin indirectly tells us about the gas-dynamical properties of galaxies and the universe on large scales,” he says.
According to Coughlin, this study paves the way for high-cadence monitoring (when many observations are taken in a short amount of time) to have the potential to reveal fundamental properties of black holes if they can be detected early on.
“New technology like the Vera C. Rubin Observatory will allow us to probe deeper into the universe than ever before,” says Coughlin. “We hope that this study offers justification for rapid X-ray follow-up of more tidal disruption events. If we can achieve this, then ideally, we can start to probe the spins of black holes through tidal disruption events.”
This research was funded, in part, by NASA and the European Space Agency.
Portions of this article have been adapted from a press release by Jennifer Chu, MIT News