A&S Physicists Help Restart Large Hadron Collider
Syracuse is U.S. host university for CERN's Large Hadron Collider beauty (LHCb) experiment
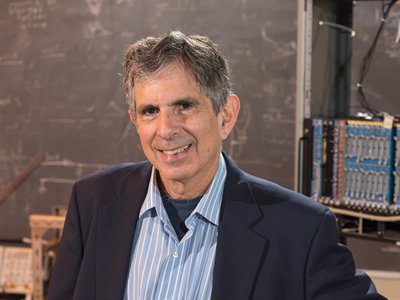
Physicists in Syracuse University’s College of Arts and Sciences are participating in the restart of the Large Hadron Collider (LHC), the world’s largest, most powerful particle accelerator.
The High-Energy Experimental Physics Group, led by Distinguished Professor Sheldon Stone, has been splitting time between Syracuse and CERN in Geneva, Switzerland. Home to the LHC, CERN is the highest energy particle physics and accelerator laboratory in existence.
After months of winter hibernation, the LHC has resumed smashing beams of protons together, in attempt to recreate conditions of the first millionth of a second of the universe, some 13.9 billion years ago.
“The faster the beams, the more energy they generate on impact—energy that is sometimes converted into heavy particles not usually produced by nature,” Stone says. “The debris from these collisions tells us a lot about the building blocks of matter and the forces controlling them.”
Syracuse is the U.S. host university for CERN’s Large Hadron Collider beauty (LHCb) experiment, involving more than 800 scientists from 16 countries. In this capacity, Stone and his colleagues are assisting with the development of detector technology for future upgrades of the LHC and its experiments.
Across the Atlantic, the LHC is working around the clock for the next six months. The machine is expected to generate approximately 2 quadrillion high-quality proton collisions—six times more than in 2015 and almost as many during its first three years of operation from 2009 to 2012.
Whereas 2015 was a recommissioning year for the LHC, 2016 should witness the delivery of the maximum amount of data to the LHCb and other CERN experiments, all of which are redefining physics beyond the Standard Model.
"Most experiments discover particles by directly producing them and measuring their byproducts," Stone says. "LHCb relies on precision measurements of rare processes, and compares how closely the theoretical predictions match the experimental results."
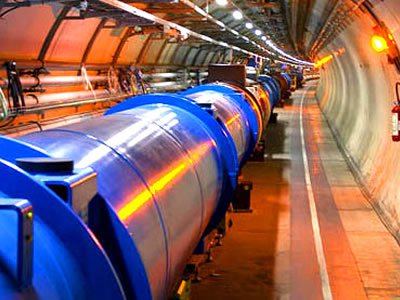
The LHC produces subatomic fireballs of energy, which morph into the fundamental building blocks of matter. The four particle detectors located on the LHC’s ring at CERN enable scientists to record and study the properties of these building blocks and to look for new particles and forces.
“The LHC accelerates protons through an enormous vacuum system, 17 miles in circumference. The protons are guided by powerful superconducting electromagnets that, in turn, are cooled by liquid helium,” Stone says. “Particles normally travel in straight lines, but the magnetic field causes them to curve. By examining the curvature [of the charged particle], we can calculate the particle’s momentum and, along with the other detection elements, establish its identity."
Between 2010-13, the LHC produced collisions in which each proton had eight tera-electron volts (TeV, or trillion electron volts) of energy. In 2015, after a two-year shutdown, the collision energy climbed to 13 TeV. “This increase in energy enables us to explore a new realm of physics that previously has been inaccessible,” Stone says.
The LHC came into public view in 2012, when scientists proved the existence of the Higgs boson, the so-called “God particle," which is 100 times more massive than the proton and can transmit forces. (Until then, the Higgs boson was the only fundamental particle predicted by the Standard Model that had not been observed.) Since then, Team Syracuse has helped advance the field of “new physics” with their discoveries, including two never-before-seen five-quark particles.
“So far, the Standard Model seems to explain matter, but we know there has to be something beyond the Standard Model,” says Denise Caldwell, physics director of the National Science Foundation. “This potential ‘new physics’ can be uncovered only with more data [from the current] LHC run.”
Among the unsolved problems of physics, which scientists hope the LHC can answer, involve the existence of gravity and dark matter (a type of matter that interacts with the visible universe through gravity) and why matter prevailed over antimatter during the formation of the early universe.
“The new LHC data will help us verify the Standard Model’s predictions, while exposing new theoretical subatomic processes,” says Stone, adding that an enormous amount of data, stemming from billions of collisions, is needed to measure well-known Standard Model processes. “Any significant deviations could be the first step toward ‘new physics.’”