Rock-Solid Data
EES Researchers Traverse to Remote Locations to Probe Earth’s Geologic History
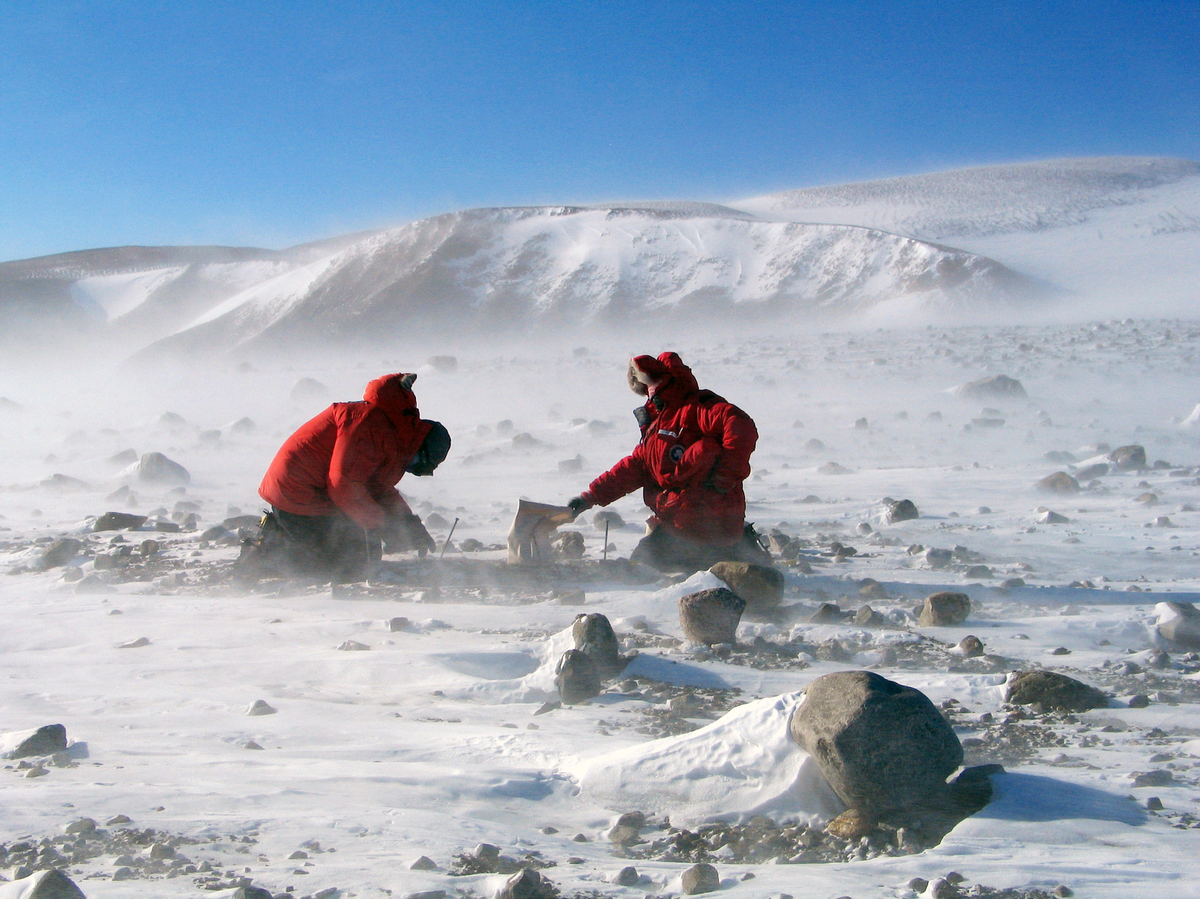
Researchers collecting boulders from Milan Ridge, Antarctica during a recent expedition. (Photo courtesy of John Goodge)
Whether investigating landslide risks in the high elevations of the Argentine Andes or exploring the puzzling history behind a mountain range in the frozen tundra of Antarctica, researchers in the Department Earth and Environmental Sciences (EES) travel the world to learn how the geography of our Earth has come to be. Their innovative research is uncovering important answers about the planet’s history in an effort to determine how global warming and climate change may affect humanity in the future. Two recent studies by EES Professors Gregory Hoke, Paul Fitzgerald and their collaborators utilized sediment and rock to spotlight the geographical evolution of two remote locations on planet Earth.
Study: Unlocking Mysteries in the Argentine Andes
Study: Discovering the Tectonic History of Sub-glacial Antarctica
Unlocking Mysteries in the Argentine Andes
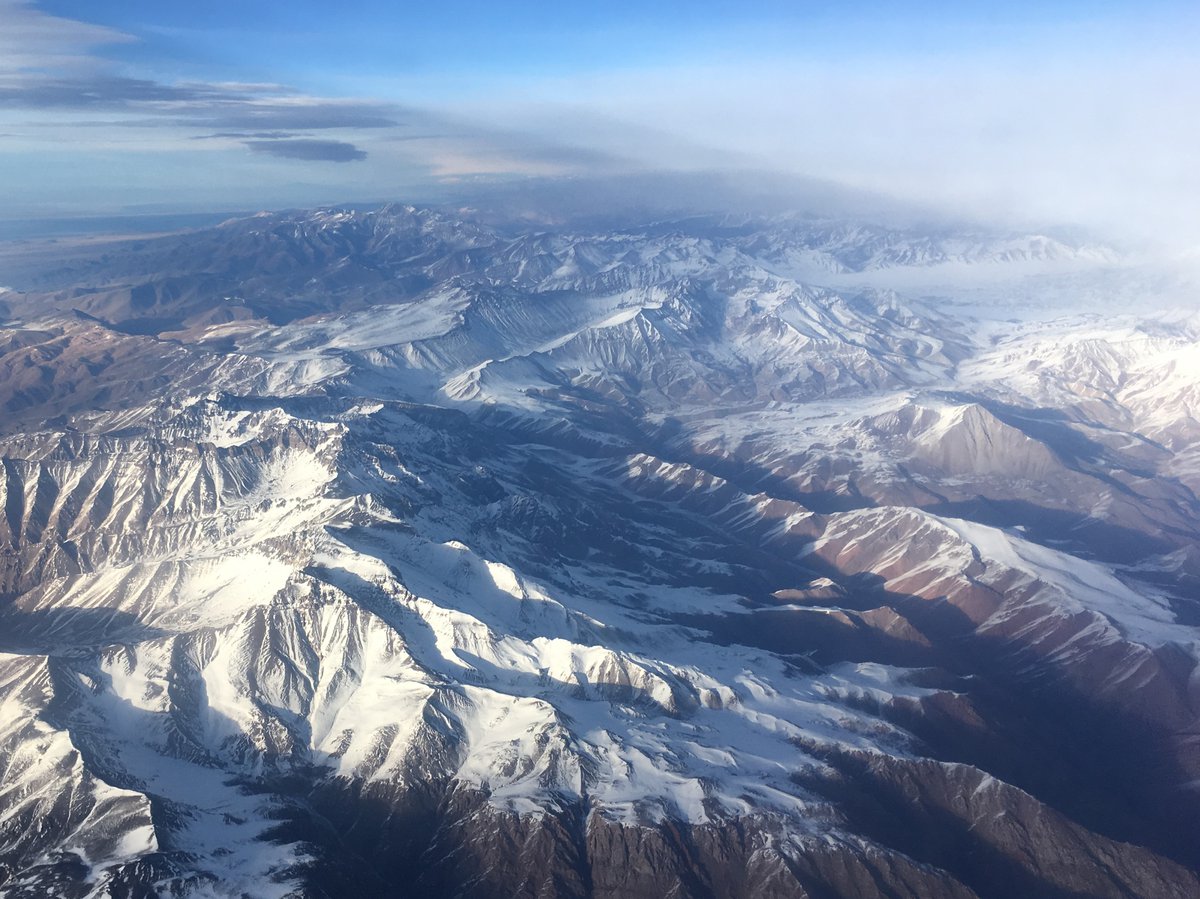
Aerial view of the study region in the Argentine Andes. (Courtesy: J.R. Slosson)
Every second the Earth is bombarded by vast amounts of cosmic rays - invisible sub-atomic particles that originate from things like the sun and supernova explosions. These high-energy, far-traveled cosmic rays collide with atoms as they enter Earth’s atmosphere and set off cascades of secondary cosmic rays. When secondary cosmic rays penetrate the upper meters of Earth’s surface, they turn elements in minerals, like oxygen, into rare radioisotopes (or “cosmogenic radionuclides”) including beryllium-10 (Be-10) and carbon-14 (C-14). Scientists can then study the variations in concentrations of these cosmogenic nuclides to estimate how long rocks have been exposed at the Earth’s surface. This in turn allows researchers to gain a better understanding of planetary processes, such as rates of erosion—from nothing more than a kilogram of river sand.
Gregory Hoke, the Jessie Page Heroy Professor and department chair of Earth and environmental sciences at Syracuse University’s College of Arts and Sciences, J.R. Slosson, a postdoctoral researcher at UMass Amherst who received a Ph.D. from Syracuse University, and Nat Lifton, associate professor of Earth, atmospheric and planetary sciences at Purdue University, recently co-authored a study published in Geophysical Research Letters analyzing cosmogenic radionuclides in samples from the Argentine Andes.
The goal of the project was to document the amount of time material resides on the hillslopes in the Andes Mountains relative to the overall erosion rate of the river basin. This information is critical to helping scientists identify landslide risks and understand how climate change will impact the dynamics of material transport on hillslopes as regions get wetter or drier.
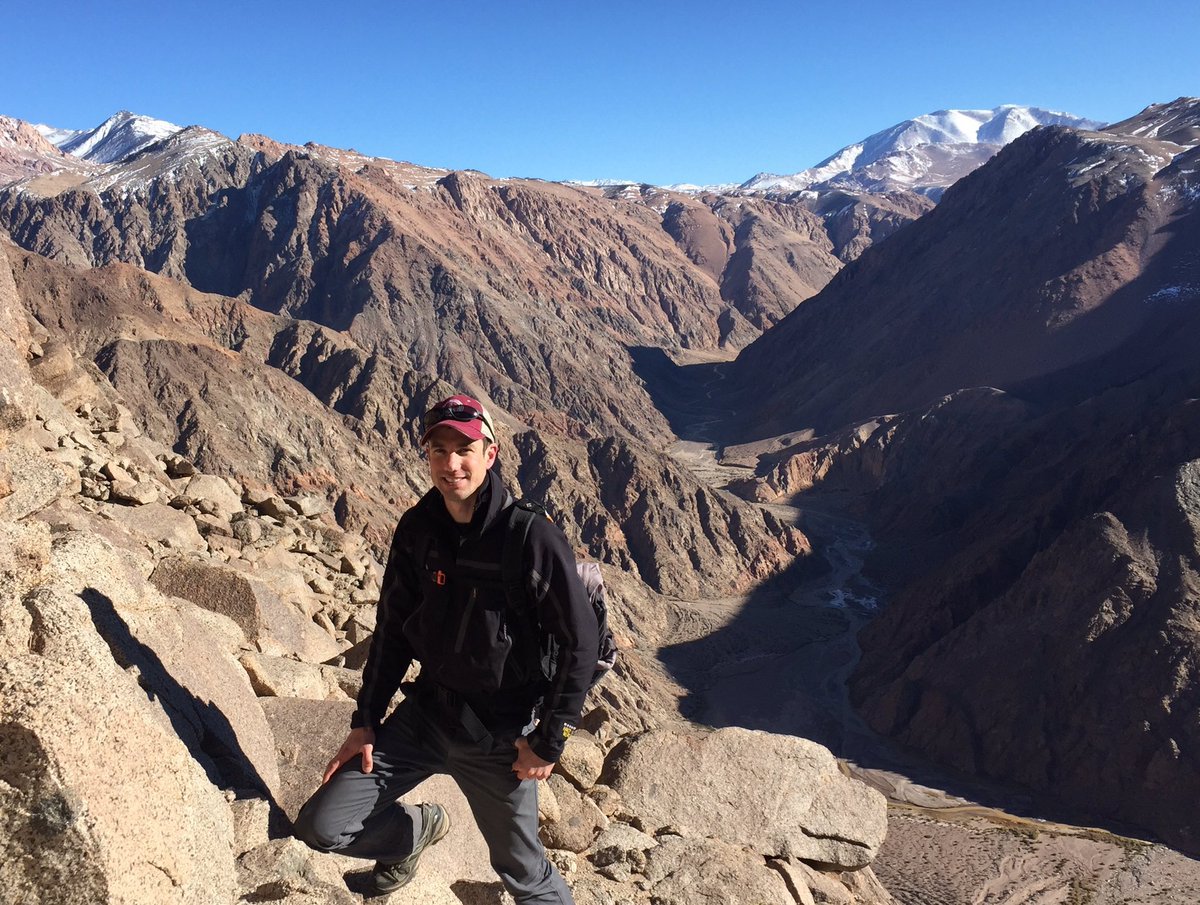
J.R. Slosson collecting a sand sample in Argentina's San Juan province. (Courtesy: J.R. Slosson)
History Written in the Sand
To determine erosion rates, the team obtained samples of river sand collected at the foot of the eastern flank of the Andes Mountains in the Mendoza and San Juan provinces, located in west-central Argentina. The river sand is to be a representative, well-mixed sample of the entire catchment (or runoff area) upstream of where the sample was collected. In Hoke’s lab at Syracuse University, the sand was treated to isolate pure quartz from the other minerals present in the sample. The researchers use pure quartz because it is an optimal source for Be-10 and C-14. Splits of pure quartz were sent to the University at Buffalo and Lifton’s lab where beryllium and carbon were extracted, respectively. Subsequent measurements of C-14 were performed at Purdue University’s PRIME Lab and Be-10 was analyzed at Lawrence Livermore National laboratory to figure out concentrations of each radionuclide.
A Tale of Talus
The highest non-volcanic peaks in the Andes are located between Santiago, Chile and Mendoza, Argentina. The river basins that drain the high Andes span 5,000 m (16,500 ft) in elevation and their hillslopes are lined with accumulations of rocky debris known as talus and scree.
Because Be-10 and C-14 are produced proportionally but decay at vastly different rates, the cosmogenic radionuclide concentrations within a sample reveal the rate at which sediment is produced from bare rock surfaces (Be-10) and the time it takes to travel down hillslopes through landslides (C-14). As sediment is mobilized and buried through landsliding, the rate of production of both isotopes diminishes, but because C-14 decays 1,000 times faster than Be-10, their proportionality changes rapidly. This change in proportionality allowed the authors to apply a statistical model to determine the average duration of time it takes material to travel down talus slopes.
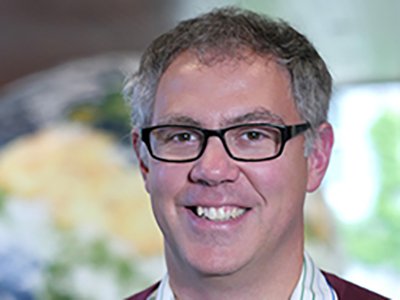
EES professor Gregory Hoke co-authored a study investigating how long material resides on hillslopes in the Andes Mountains.
According to author Gregory Hoke, this is one of the first studies to use the combination of Be-10 and C-14 to show the long-term average rate of sediment generation and the time and process it takes to move down to and through the rivers, giving a broader picture of the factors involved.
While the concentration of Be-10 came back as anticipated over the long timescale, they found that C-14 was much lower than anticipated, meaning that sediments eroded from the high mountain watersheds were shielded from cosmic rays for at least 7 to 15 thousand years. The authors explain that temporary storage in talus slopes best explains the lower concentration of C-14 relative to Be-10.
“This study shows that it is possible to fill an important gap in the observational timescale using the C-14/Be-10 pair that brings to life what really happens on the hillslopes,” says Hoke.
With the risk that landslides pose to humans and infrastructure, J.R. Slosson says their results indicate that C-14 can be significant in unraveling sediment transport dynamics going forward, and potentially help predict where future landslides might occur. He explains, “utilizing C-14 along with Be-10 provides a new window into the complexity of sediment transport in mountain settings and can provide a backdrop for evaluating contemporary changes in earth surface processes.”
Discovering the Tectonic History of Sub-glacial Antarctica
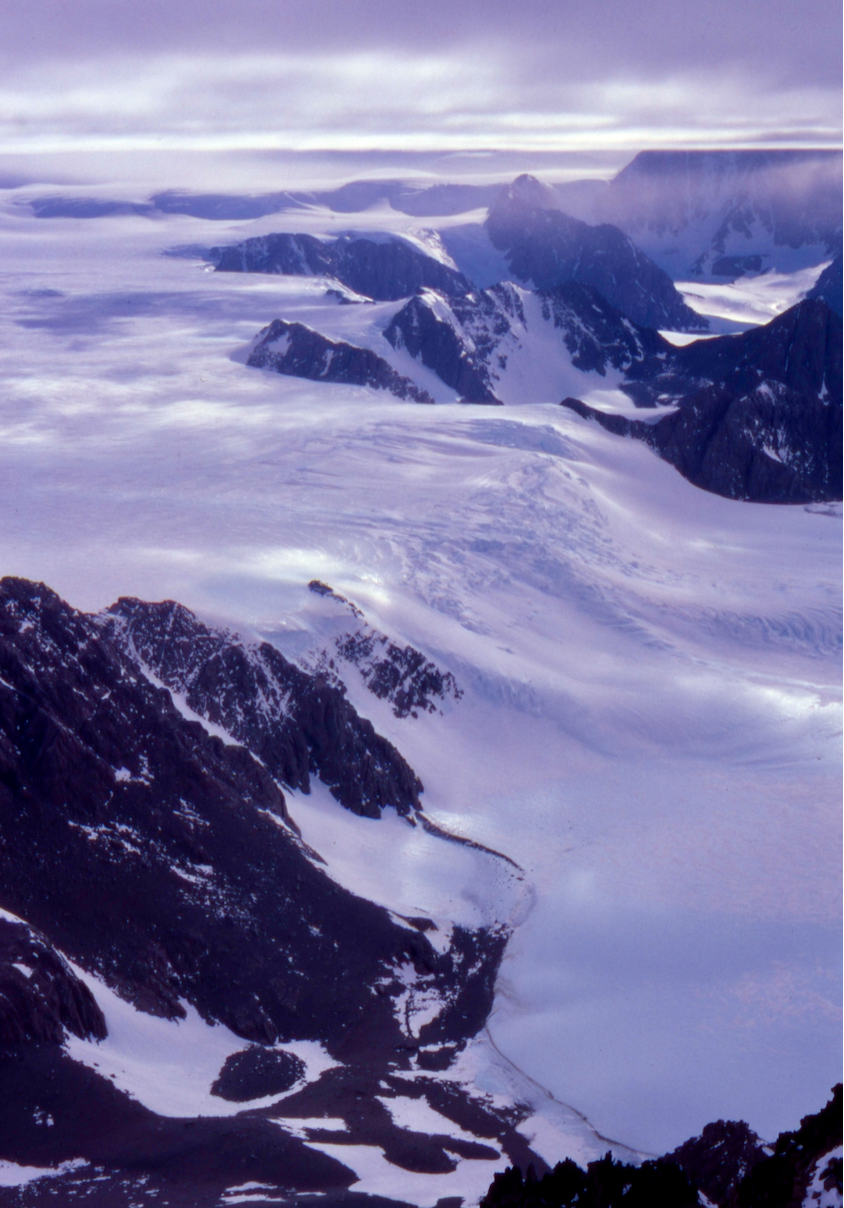
Ice from the East Antarctic Ice Sheet flowing through the Transantarctic Mountains. Photo taken from the summit of Mt. Borcik in the Scott Glacier region of the Transantarctic Mountains at 86°S. (Photo by Paul Fitzgerald)
A trove of ancient rocks collected from glacial moraines has literally revealed the deep story of one of the most underexplored environments on the planet—the rocks and mountain belts hidden beneath the East Antarctica Ice Sheet. Before this study, scientists had only the vaguest idea of when, how and why the mountains and landscapes now buried under the world’s largest ice sheet had formed.
“These mountains and landscapes have been buried under miles of ice for the past 14 million years,” says EES Professor Paul Fitzgerald. “They are much more remote than Mount Everest or the deepest part of the ocean.”
Now, some of that inaccessible region’s tectonic mysteries have been solved. In a study recently published in Nature Communications, first author Fitzgerald and second author John Goodge, a professor emeritus of geological sciences at the University of Minnesota Duluth, used innovative rock sampling and radiometric dating on those rocks to reveal the history of central East Antarctica over the past half-billion years.
Mysterious Mountains
The researchers selected granite boulders ranging in age from 1 to 2 billion years old, knowing that rocks of this age do not occur anywhere else in Antarctica and must therefore come from well under the ice sheet, perhaps from the large and mysterious Gamburtsev Subglacial Mountains. This is because as mountains erode, glaciers—slow-moving rivers of ice—transport the boulders to fields of deposited rock and sediment, called glacial moraines, near the edge of the continent.
Scientists have long puzzled over the Gamburtsev Subglacial Mountains purely because they are completely buried under the ice, and no one knows what rock types make up the range. Recent sub-ice images of the range suggest a non-volcanic origin, but if not volcanism, then what tectonic forces were responsible for its formation? Knowing when the mountains formed means researchers can start to solve this puzzle.
But how do you determine when a mountain belt formed, yet alone one that is completely buried under the ice? By determining the low-temperature cooling history of the rocks. And that’s where complementary expertise comes into play.
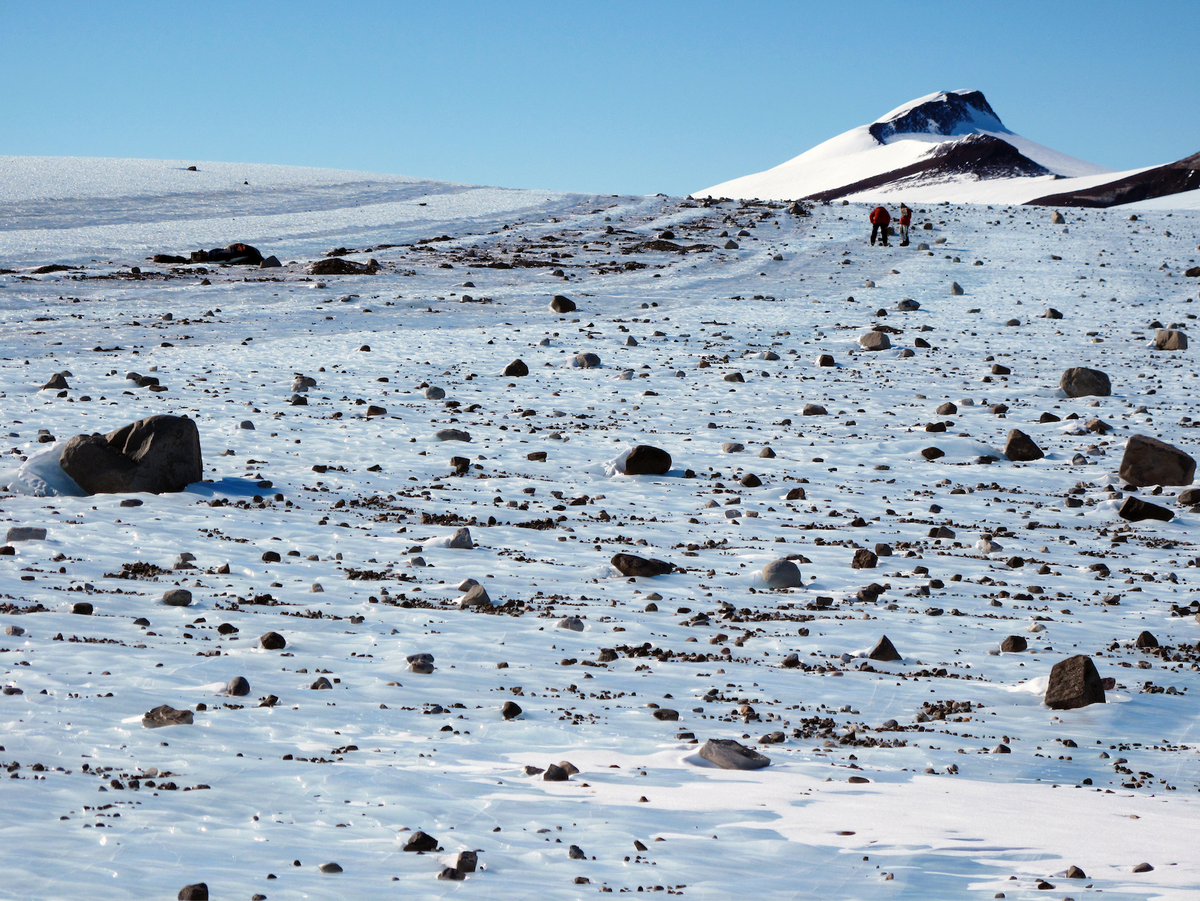
Glacial moraine at Lonewolf Nunatak. (Photo courtesy of John Goodge)
Fitzgerald, a New Zealand native, and Goodge, an American, have been good friends since meeting as graduate students in the Transantarctic Mountains in 1986. But they had never worked together. Now they had a chance to do just that. Goodge had collected the boulders and Fitzgerald had the expertise with thermochronology. Both have been working on Antarctic geology for decades.
When mountains are formed, they are uplifted and form high topography. Due to erosion, rocks are then exhumed towards the surface. As rocks are exhumed, they cool. Determining when and how fast rocks cool helps narrow down when in geologic time the mountains formed. Geologists use thermochronology—analyzing the time-temperature history of rocks through radiometric dating–to understand the cooling history. The relatively large size of the boulders allowed researchers to analyze the boulders using a variety of radiometric methods.
The Findings
The results showed that the interior of East Antarctica had experienced three major periods of rapid cooling which are due to major tectonic events. First, the supercontinent Gondwana formed due to continent-continent collision about 500 million years ago. Second, the Gondwana supercontinent started to break up about 180 million years ago. Finally, a high-elevation plateau started to collapse about 100 million years ago, associated with formation of a rift-system between East and West Antarctica. All in all, these conclusions actually make good geologic sense.
These are still very early days in understanding the geologic puzzles that are concealed by the vast mass of the East Antarctic Ice Sheet. What stands out for Fitzgerald, though, is the excitement of discovery. “We were sampling a place that we knew very little about. For us, this was like having rocks to study from the moon or Mars.”
Learn more about the Department of Earth and Environmental Sciences.
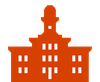
Further Reading
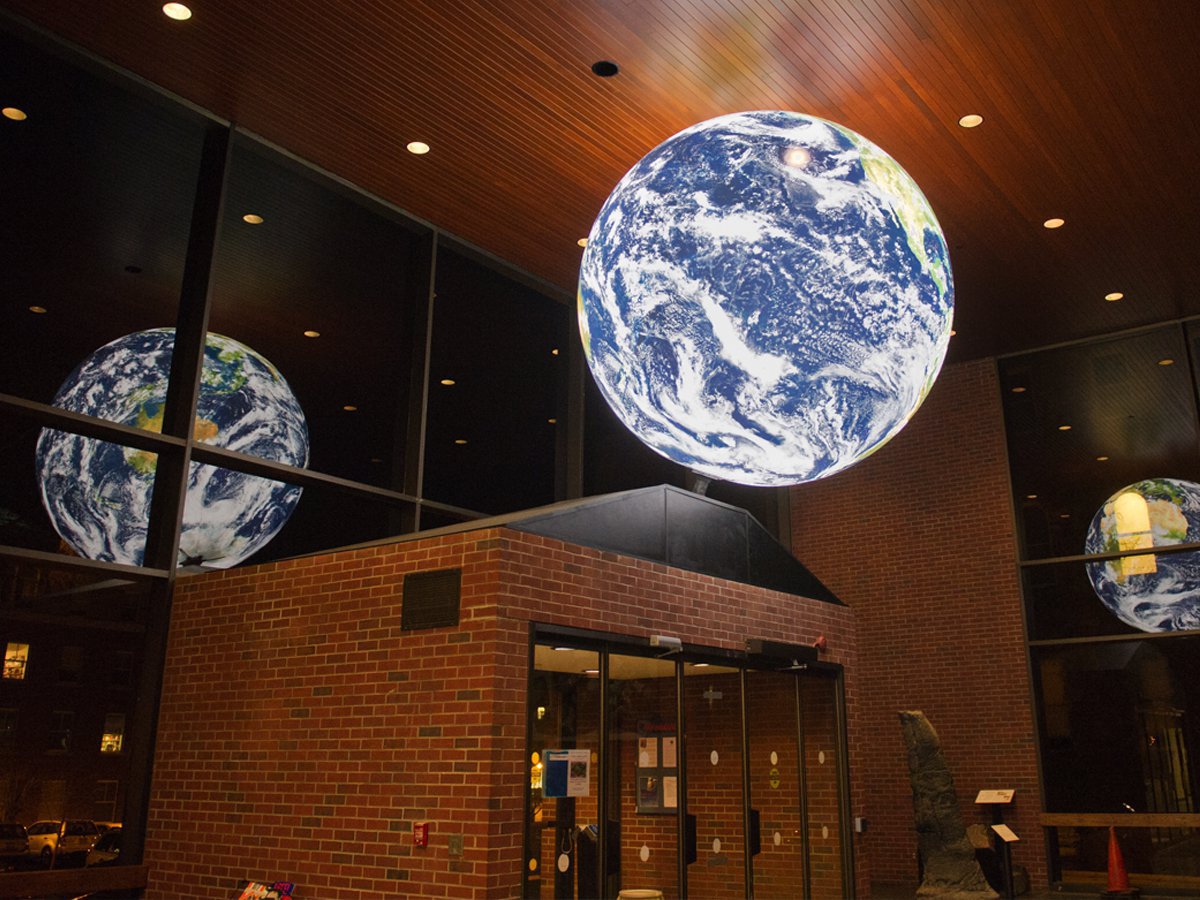
Department of Earth and Environmental Sciences
The study of Earth sciences combines experiential learning, interdisciplinary study and a culture of innovation and discovery. Learn more about the Department of Earth and Environmental Sciences.
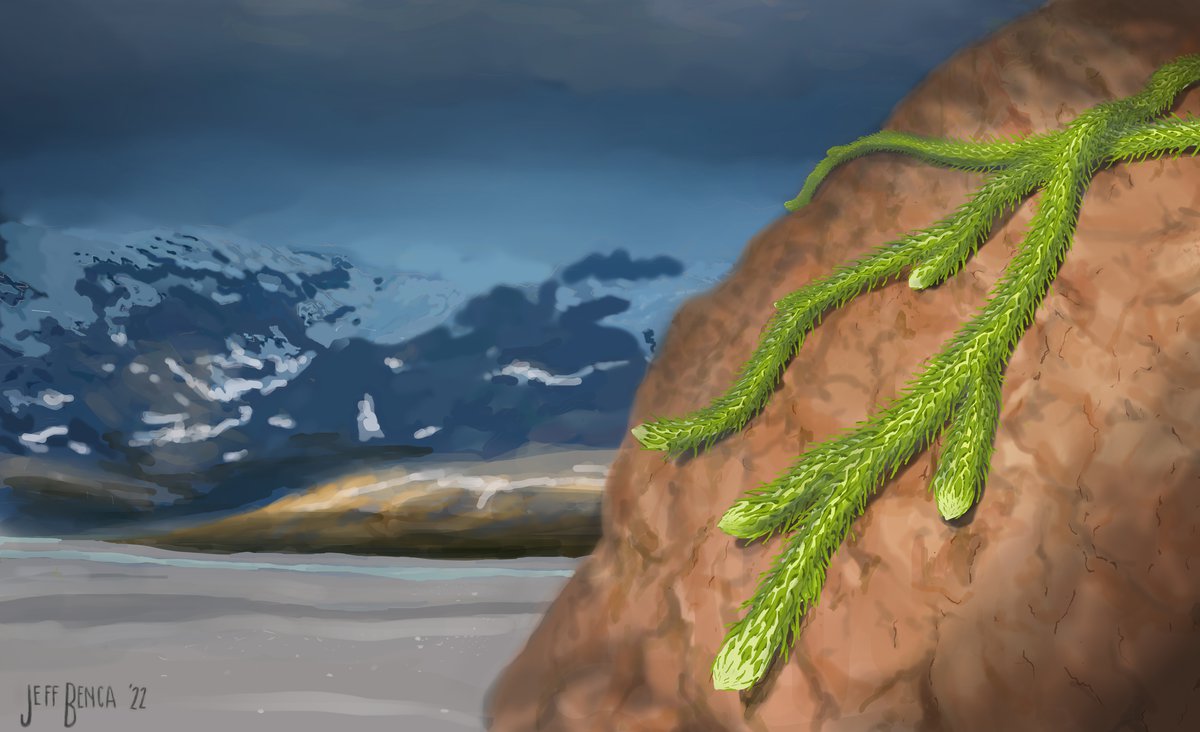
Uncovering Ancient CO2 Levels
Ever wonder what our climate might be like without trees? EES Professor Christopher Junium and a team of researchers recently discovered that the emergence of large forests may not have played as important of a role in decreasing carbon dioxide as previously thought.